Majorana 1: The First Topoconductor Chip and a New Era in Semiconductor Physics
- jenniferg17
- Apr 7
- 3 min read
Updated: Apr 9

Microsoft's unveiling of the Majorana 1 chip on February 19, 2025, marks a significant milestone in the evolution of quantum computing. This processor introduces a novel Topological Core architecture, leveraging groundbreaking topoconductor materials to enhance qubit stability and scalability. This article explores the novel topoconductor materials, the qubit architecture of Majorana 1 and its implications for future quantum applications.
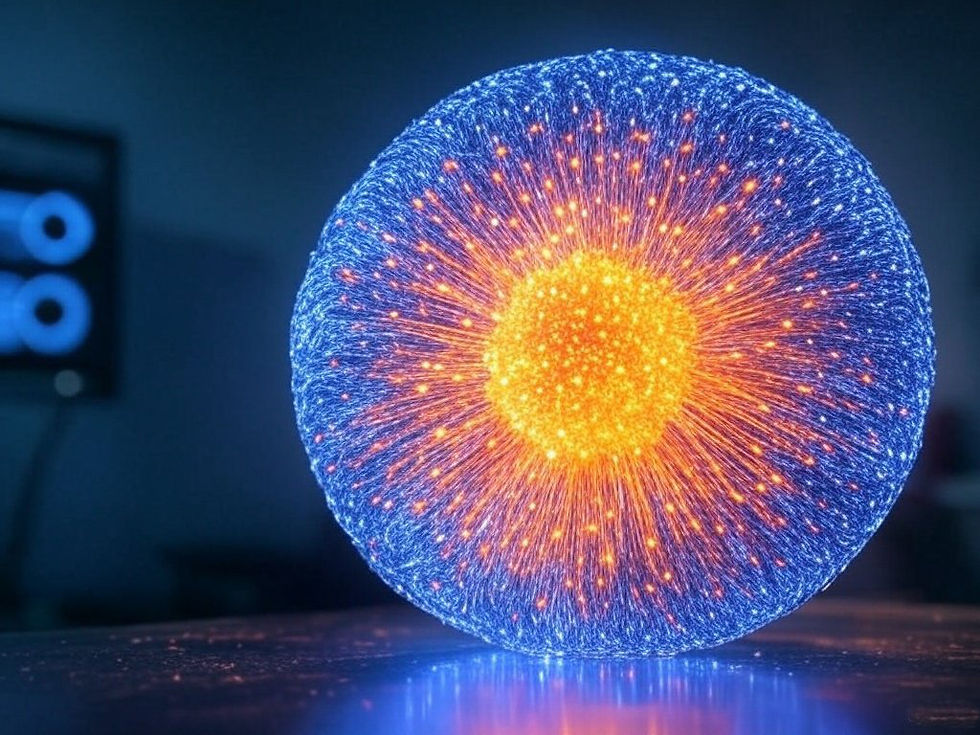
1. Introduction to Topoconductors
At the heart of Majorana 1 lies the topoconductor, an evolution of topological insulators and superconductors, that facilitates the creation and stabilization of Majorana zero modes (MZMs). These quasiparticles are essential for constructing topological qubits, which offer enhanced robustness against environmental disturbances.
Key Properties of Topoconductors:
Material Composition:
The "Majorana 1" topoconductor is a hybrid structure comprising indium arsenide (InAs) and aluminum (Al). This combination fosters conditions conducive to the emergence of MZMs. Indium arsenide provides strong spin-orbit coupling, while aluminum induces superconductivity via proximity effects, creating an environment suitable for MZM formation.
Operational Conditions:
Ultra-Low Temperatures:
Majorana zero modes require cryogenic temperatures (approximately 10 millikelvin) to maintain superconducting properties and suppress quasiparticle poisoning. At these temperatures, thermal excitations are minimized, preserving the coherence of the superconducting state essential for MZM stability. Quasiparticle poisoning, which involves unwanted quasiparticles disrupting the system, is significantly reduced at ultra-low temperatures, thereby enhancing the reliability of MZMs.
Magnetic Field Conditions:
A precisely tuned magnetic field (typically around 0.1 to 0.2 Tesla) is applied to break time-reversal symmetry, a necessary condition for MZMs to emerge. Breaking this symmetry transitions the system into a topological superconducting phase, facilitating the formation of MZMs. However, the magnetic field must be carefully calibrated; excessive field strengths can close the superconducting gap, destabilizing the MZMs.
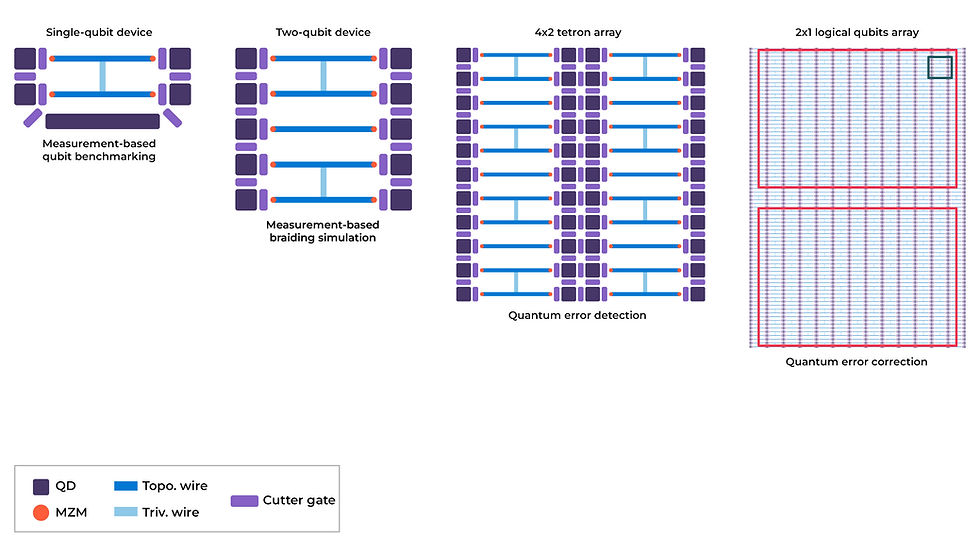
2. Architectural Innovations in Majorana 1
Majorana 1 introduces several architectural advancements designed to enhance qubit performance and scalability:
Qubit Configuration:
Current Qubit Count: The chip integrates 8 topological qubits, each constructed using an 'H'-shaped configuration of indium arsenide and aluminum nanowires. The 'H '-shaped nanowire layout consists of four junctions where Majorana modes emerge at each edge, enabling fault-tolerant qubit operations.
Scalability Roadmap: The architecture is engineered with a threshold believed necessary for practical, large-scale quantum computations. Current research suggests that a fault-tolerant quantum computer may require millions of logical qubits and Majorana 1 represents an early-stage implementation with potential for scalability.
Error Mitigation and Stability:
Topological qubits derived from MZMs exhibit inherent resilience to environmental noise and decoherence, unlike conventional superconducting qubits, which require surface code error correction, topological qubits rely on the braiding of Majorana zero modes, making them inherently protected against local perturbations. This intrinsic stability reduces the necessity for extensive error correction protocols, thereby enhancing computational efficiency.
3. Comparative Analysis: Majorana 1 vs. Conventional Quantum Chips
To contextualize Majorana 1's advancements, a comparison with traditional superconducting qubit-based quantum processors is presented:
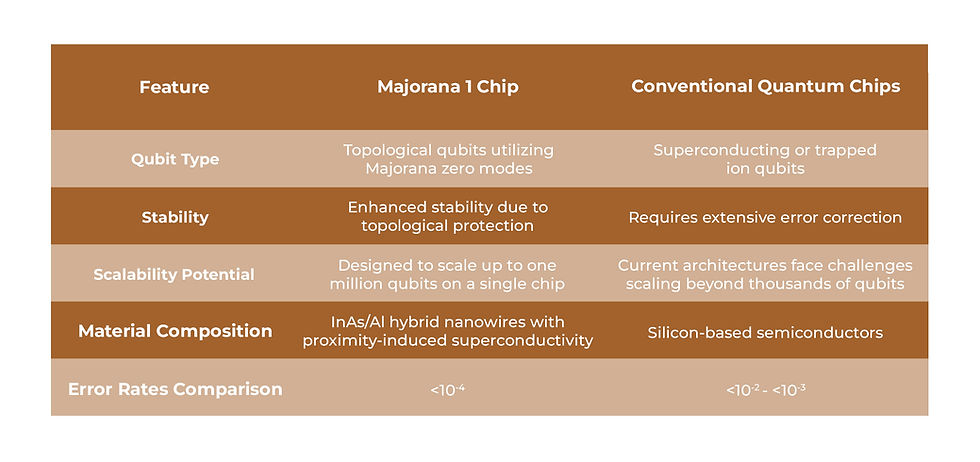
4. Implications for Quantum Computing
The integration of topoconductors in Majorana 1 signifies a transformative leap toward practical quantum computing:
Enhanced Computational Power: The potential to scale up to a million qubits enables the resolution of complex problems currently beyond the reach of classical computers. For example, a million-qubit quantum computer could simulate electron interactions in high-temperature superconductors, advancing energy-efficient technologies, or model complex molecular interactions, accelerating drug discovery by precisely predicting protein folding and enzyme reactions—tasks that classical computers struggle to handle due to the exponential complexity of quantum states.
Energy Efficiency: Unlike superconducting qubits that require active error correction, Majorana qubits have passive error resistance, reducing the need for redundant qubits and power consumption.
Broader Applications: From cryptography to material science, Majorana 1's advancements pave the way for quantum solutions across diverse industries.
In summary, Microsoft's Majorana 1 chip, underpinned by topoconductor technology, addresses critical challenges in qubit stability and scalability. This innovation not only propels quantum computing forward but also opens new avenues for practical applications in solving complex, real-world problems.
At McKinsey Electronics, we’re dedicated to ensuring that revolutionary products like the Majorana 1 chip are readily available to engineers across the ATME region—empowering you to redefine the future of quantum computing. Stay updated on the latest developments in the semiconductor industry and visit subscribe to our monthly newsletter.